توضیحات
Abstract
An analytical method called biosensing uses biological analytes, such as DNA, RNA, or protein, to detect the beginning or progression of many acute and chronic disorders. Biosensing devices keep track of a particular biological analyte’s activity in a complex illness environment. A bioreceptor, a transducer, and an electrical component that amplifies the signal make up the three main parts of a biosensor system. A biological reaction is produced when the bioreceptor perceives or interacts with a particular bioanalyte, and the transducer measures this response. The biosensor’s electrical system further processes, displays, and amplifies the produced signals. The entire procedure is so quick and precise that it may be utilized as a point-of-care tool in real-time. The chosen bioreceptor affects the biosensor’s specificity. The bioreceptor interacts with the bioanalyte either through an affinity-based or catalytic process. A physiochemical change in electric charge, a visible calorimetric reaction, or a fluorescence response are the results of the contact. A peptide or antigen is highly specifically and strongly bound by antibody-based bioreceptors. The amide, ester, or thiol linkages are often used to attach the bioreceptor antibody to the surface. However, utilizing antibodies has certain drawbacks, such as limited stability and expense. Additionally, the environment needed for the antibody-antigen interaction was extremely complex; even a slight change in pH or temperature might prevent an interaction. Although the antibody and antigen binding is typically active, it can be interfered with by an organic environment, chaotropic substances, and ultrasonic waves. Enzymes that were utilized as bioreceptors, on the other hand, were responsible for the catalytic-based interactions. Covalent bonding, cross-linking, or trapping are all effective ways to immobilize the enzymes on the membrane. Because it is more selective than antibody-based bioreceptors, the enzyme–substrate interaction is superior. Enzyme utilization has several benefits, including greater turnover, selectivity for a subset of bioanalytes, and adaptability for different transducers. A higher overall 5-year survival rate can be attained with early detection of serological indicators for ovarian cancer. A single biomarker’s finding, meanwhile, does not necessarily indicate how ovarian cancer is biologically. To detect blood biomarkers such as cancer antigen 125 (CA-125), 2-microglobulin (β2-M), and Apo-lipoprotein A1 (ApoA-I), Pal et al. developed a fluorescent multiplexed magnetic nanoparticle-antibody conjugate (MNPs-Abs). In the early stages of ovarian cancer, CA-125 is directly produced by the cancer cells, and its amount in the blood increases dramatically. However, its level is raised in premenstrual patients due to a few other circumstances, which reduces its specificity. Because MHC-I is downregulated in cancer cells, increased levels of β2-M in serum are likewise linked to ovarian cancer. All nucleated cells have β2-M displayed as an antigen on their surface by MHC I through non-covalent contact. The movement of cholesterol from peripheral cells to the liver depends on ApoA-I. Its level was discovered to be lower in the serum of those with ovarian cancer. They used the EDC/NHS blocking chemistry to functionalize the MNPs, ionizing anti-antibodies (anti-abs) quickly and then slowly covalently connecting them. The resulting MNPs-Abs demonstrated a respectable sensitivity and specificity of 94% and 98%, respectively, to distinguish between healthy individuals and patients with early-stage ovarian cancer. Han et al. published a study on magnetic-fluorescent iron oxide carbon hybrid nanoparticles (MCNP) for the early stage cancer detection. The authors described a straightforward, one-step synthesis of MCNP that had a high quantum yield and outstanding photostability. In a multicomponent reaction including citric acid, EDTA, citric acid, FeCl3, and FeCl2.4H2O in diethylene glycol, the MCNP was created. To precisely detect breast cancer cells, anti-CD44 polyclonal antibodies were used to manufacture the MCNP, which had a high quantum yield of 58.4%. Comparing the resulting smart MCNP to pure IOPN contrast agents, the T2 relaxivity ratio of the latter was improved. In comparison with the C44 breast cancer cell, 4T1, the formulation demonstrated an improved and specific dual fluorescence and MRI contrast agent. Additionally, the breast cancer cells effectively absorbed MCNP intracellularly, as demonstrated by transmission electron microscopy. With a sensitivity to finding as little as 100 breast cancer cells in a heterogeneous population, the newly developed CD44-MCNP was capable of differentiating between the CD44-positive (4T1) and CD44-negative cell populations. For the quick identification of cancer in subjects, electrochemical approaches have become popular. The cells linked to the electrode create electrons as a result of numerous ongoing biological processes, which are then transmitted to the electrode and can be employed as biosensors. PEGylated arginine functionalized MNPs-based electrochemical impedimetric biosensor for the detection of cervical cancer was reported by Chandra et al. First, dried methanolic solutions of L-arginine were combined with di(ethylene glycol)ethyl ether acrylate to create PEGylated arginine. Ball-tube distillation was used to further purify the product once the solvent was eliminated. The PEGylated Arginine was used to stabilize the MNPs produced by the co-precipitation technique, and the resulting PA-MNPs were measured to be in the ~9 nm range. HeLa cells were next added to the PA-MNPs for incubation, after which IgG antibodies were added. The electrochemical impedance studies with various HeLa cell concentrations after electrode modification demonstrated a sensitive detection of up to 10 cells/ml. According to the study, PA-MNPs speed up the transmission of electrons, improving the selectivity of the biosensors. The production of reactive oxygen species (ROS) is regarded as a typical by-product of metabolism and is essential for homeostasis and cell signaling. But it also has a significant impact on the development and spread of many acute and chronic illnesses, including cancer. Therefore, a viable method for identifying various illnesses is the measurement of ROS in biological fluid. The first magnetic relaxation switching (MRSw)-based biosensor for measuring ROS was described by Lee et al. MRSw has been effectively employed for the sensing of a variety of biomarkers and takes advantage of the target-based aggregation and disaggregation of MNPs. The researchers used straightforward sonication to create PEGylated bilirubin-coated SPIONs (PEG-BR@SPIONs). The PEG-BR@SPIONs were loaded with a fluorescent near-infrared dye to guarantee fluorescence-based ROS detection. When subjected to an in vitro model of ROS, the extremely colloidal stable PEG-BR@SPIONs agglomerate reduces the strength of the T2-weighted MRI signal. In comparison with ROS-unresponsive control NPs, the created MRSw-based biosensor demonstrated effective internalization in lipopolysaccharide-activated macrophages. In a clinical setting, the PEG-BR@SPIONs biosensor was able to detect the level of ROS in the blood samples via a clear decrease in T2 weighted MRI signal and enhanced fluorescence signal. According to the study, PEGylated Bilirubin-coated Iron Oxide NPs can be used as a dual-functional sensor for the quick and accurate detection of ROS in a variety of illness conditions. A methylated DNA base called 5-methylcytosine (5-mC) controls the expression of genes, which in turn affects a number of biological functions. The methyl group is joined to the cytosine’s fifth carbon by the enzyme DNA methyltransferase. The 5-mC is a crucial component of numerous biological processes, including cellular development, aging, and carcinogenesis. The ten-eleven translocation enzyme sequentially oxidizes 5-mC to produce demethylated 5-carboxylcytosine. All of the intermediate products of the demethylated pathways, including 5-mC, are essential regulators of carcinogenesis and serve as a crucial marker for the development and progression of cancer. Pingarron and colleagues employed immunomagnetic beads with selective nucleic acid hybridization to evaluate the methylated base from blood, cancer cells, and tissue samples to specifically sense the 5-mC in the tumor suppressor gene. Here, the O(6)-Methylguanine-DNA-methyltransferase (MGMT) tumor suppressor gene’s promoter region is sensed by the researchers using an immuno-DNA-based technique. The HOOC-magnetic beads (MBs) were functionalized with the anti-5-mC antibody using EDC/Sulfo-NHS chemistry. The 5-mC-methylated ssDNA sequence is recognized and immobilized by the monoclonal antibody. The methylation ssDNA’s flanking area is hybridized with artificial biotinylated complementary DNA probes, which are then detected by streptavidin-HRP and result in an amperometric measurement. According to the researchers, the amperometric biosensor they designed has a LOD of 1.2 pM and a dynamic detection range of 3.9–500 pM. The same research team later created a more affordable and simple technique for the simultaneous detection of 5-mC and 5-Hydroxymethyl-Cytosines (5-hmC), an intermediate product of demethylated pathways. Here, the group created a sandwich-type immunoassay using DNA probes and antibodies for the PCR-independent detection of methylated DNA bases at both the global and gene-specific levels. The 5-mC and 5-hmC bases in the promoter regions of two tumor suppressor genes, RASSF1A and MGMT, were effectively analyzed. Immune-MBs and DNA probes are coupled without the need for a PCR, and the outcome is electrochemical detection at screen-printed carbon electrodes (SPCE). The resulting bio-tool enables rapid, highly reproducible, selective, and simultaneous detection of the methylated base in less than 90 min. Nanozymes have proven to be a quick, affordable, and accurate way to find methylated bases in cancer samples. Mesoporous iron-oxide NP (MIO) with peroxidase activity was created by Bhattacharjee et al. to detect 5-mC in CRC cell lines. The dextran-modified MIO was first functionalized overnight at 4 °C using an anti-5-mC antibody. The methylated base (5-mC) on the target ssDNA adsorbed on the surface of a naked screen-printed gold electrode (SPGE) was recognized using the antibody-functionalized MIO. 3,3′,5,5-tetramethylbenzidine (TMB) is oxidized by the peroxidase activity of the MIO bound to the surface of SPGE in the presence of H2O2, which leads to the calorimetric and amperometric detection of the methylated base. With great repeatability and specificity (%RSD ≥ 5%), the developed biosensors successfully detect a small, i.e., 10% change in the base methylation in synthetic samples. Shaban et al. provided a second investigation utilizing the electrochemical immunosensor for the detection of tumor antigen. They used the signal-enhancing properties of several nanocomposites to build a sandwich-type sensor for tracking prostate-specific antigen (PSA) in actual samples. They started by making a magnetic IONP that had been modified with poly(3,4-ethylenedioxythiophene):poly(styrene sulfonate) [Fe3O4/PEDOT:PSS]. To begin with, they used the co-precipitation approach to create Fe3O4 MNPs. The Fe3O4 MNPs were properly cleaned before being dissolved in PEDOT:PSS solution. The produced Fe3O4/PEDOT:PSS nanocomposite was applied to a glassy carbon electrode for the immobilization of primary anti-PSA antibody coupled with biotin in order to construct immunosensors. A gold nanoparticle with a cysteamine cap (AuNPs-CysA) was coupled with the secondary antibody to speed up the electron transfer rate and boost electrochemical signal amplification. With a low limit of quantification, the developed electrochemical immuno-sensor demonstrated a broad linear range against the PSA bioanalyte. In conclusion, the immuno-sensor proved effective in detecting PSA in human plasma samples that had not been treated. For bioanalyte detection and in vitro fluorescence imaging, graphene oxide (GO) has proven successful, but its in vivo applicability is still not well understood. To improve the functioning of GO as a drug delivery agent and MRI contrast agent, Naumov and colleagues coupled it with SPION (Fe3O4 NPs). In comparison with GO, the synthesized GO-Fe3O4 was less cytotoxic toward the three tested cell lines, HeLa, MCF-7, and HEK-293 cells. Due to the superparamagnetic nature of Fe3O4 NPs, GO-Fe3O4 demonstrated high r2/r1 relaxivity ratios of 10.7 when used as an MRI contrast agent. The GO-Fe3O4 NPs were able to distinguish between diseased and non-cancerous cells because to the pH sensing ability of GO. The fluorescence pictures demonstrated that the developed NPs effectively delivered the hydrophobic medication, doxorubicin, with a 2.5-fold increased cytotoxicity. The study reported a multifunctional magnetic targeted platform with efficient hydrophobic drug delivery in vitro and an enhanced r2/r1 relaxivity ratios for in vivo MRI optical imaging. Small non-coding ribonucleic acid molecules called microRNAs are in charge of post-transcriptional gene regulation. The development of several cancer types is correlated with deregulated expression of microRNA21 (miR-21). Given that miR-21 is involved in a variety of malignancies, it is an excellent candidate to be used as a key diagnostic and prognostic marker. Numerous studies have demonstrated that the accurate grading of the disease’s various phases was made possible by the effective detection of miR-21. Islam and colleagues developed a special electrochemical sensor to accurately measure the level of miR-21 using the electrocatalytic activity of graphene oxide-loaded iron oxide (GO/IO NPs) and the signal enhancing property of ruthenium hexamine (III) chloride (Ru(NH3)6Cl3; RuHex). Here, the morphology of GO/IO NPs has been changed in a way that both enhances the NPs’ porosity and improves their surface functioning. This alteration makes it easier for magnetically separated and pure miR-21 to bind to the GO/IO hybrids put on a screen-printed electrode via graphene-RNA affinity. RuHex, which was electrostatically bound to the phosphate backbone of miR-21, was present when the adsorbed miR-21 was measured using a chronocoulometric readout. With a LOD of 1.0 fM and 10 cells, respectively, in a synthetic sample and cancer cell population, the constructed biosensor exhibits outstanding sensitivity. Further, Tian et al. created MNPs assemblies with DNA scaffolds that include substrate sequences for DNAzyme, serving as a point-of-care device for the detection of microRNAs. The DNAzyme will react with the target microRNA in the presence of it and catalyze the cleavage reaction, causing the scaffold to separate and the MNPs to fragment. A 405 nm laser-based optomagnetic sensor can measure the concentration of the disintegrating MNPs, which will be proportional to the target microRNA sequence. It’s interesting to note that the DNAzyme did not completely destroy the target sequences during the cleavage event; hence, after being released in the suspension, these target sequences cause the dissolution of several more MNPs. The resulting nanostructures showed a significant LOD of 1.5 pM and 6 pM against the target microRNA (let-7b) and target DNA, respectively. Nebu et al. create a method for bioimaging malignant cells using a magnetic nanoprobe. The magnetic iron oxide core of their formulations is surrounded by gold nanoclusters electrostatically adsorbed. Erlotinib, an FDA-approved inhibitor of the epidermal growth factor receptor (EGFR), an oncogenic marker for pancreatic cancer, was further conjugated with the gold nanoclusters. The magnetic property is provided by the iron oxide core, while the photo-stability, significant Stoke shifts, and great biocompatibility are provided by the gold nanostructures. As pancreatic cancer targeting agent, the synthesized 24 ± 2.5 nm Fe3O4@AuNCs had a negative core conjugated to a positively charged form of erlotinib. The formulation demonstrated in vitro drug release at the acidic pH, and pancreatic cancer cells that were positive for the EGFR displayed increased red fluorescence than control cells. Additionally, MTT results indicated that the formulation could kill cells through EGFR-mediated targeting, as evidenced by the fact that more EGFR overexpressing cell lines were killed. In summary, this research shows how well MNPs may be used for both treatments and diagnostics. Cancer stem cells (CSCs) have been identified by researchers as the main factor in cancer recurrence and as having a significant impact on the development and spread of the disease. CSCs are a rare bunch of cells displaying self-renewal properties, the prospective to differentiate in tumor cells, and high-level resistance to accessible chemo- and radiotherapy. The CSCs are located at the very top of the tumor’s organizational hierarchy. CSCs have many characteristics in common with healthy body stem cells, but they also have several limitations. The limitations encompass the expression of different surface markers linked to typical stem cell growth as well as the dysregulation of a number of cellular pathways. Several CSC surface markers, including CD133, SSEA-1 or CD15, CD90, TDGF1, and PODXL-1, are widely utilized to identify these cells. A CD133 cell surface marker-specific immunomagnetic nanosensor for the imaging of glioma CSCs was disclosed by Wang et al. First, a nanosensor core made of superparamagnetic g-Fe2O3 iron oxide NPs (SPIONs) with a size range of 10–15 nm was created. This core was then covered with carboxymethyl chitosan via a crosslinking process using sodium tripolyphosphate. Polyethylenimine (PEI) was used to chemically modify the NPs further, and Traut’s Reagent and sulfosuccinimidyl-4-(N-maleimidomethyl)cyclohexane-1-carboxylate were used to couple the anti-CD133 monoclonal antibodies to the PEI-SPIONs. The mAb-SPIONs was also connected with the fluorescent rhodamine B isothiocyanate (RBITC) probe for fluorescence imaging. The fluorescently tagged mAb-SPIONs exhibits high biocompatibility and is not harmful to glioma cells or CSCs, according to the in vitro tests. When treated with mAb-SPIONs, the CD133 positive CSCs displayed a bright red fluorescence and a lower MRI contrast enhancement than the non-mAb-functionalized-SPIONs in T2-weighted MRI. The study recommended creating an effective SPION that particularly targets the CSCs and may be utilized as an MR contrast agent as well as for real-time monitoring via confocal imaging. In a research, Choi and coworkers revealed a novel, MRI-based phenomena based on magnetism that may monitor interactions between biological analytes. In this study, the researchers coated the surface of silica-embedded MNPs (Zn0.4Fe2.6O4) with a T1 contrast agent, Gd (III) 1,4,7,10-tetraazacyclododecane-1,4,7,10-tetraacetic acid (Gd-DOTA). By reducing the thickness of silica NPs from 18 to 2 nm, the distance between the Gd-DOTA and MNPs was controlled. The work suggested a unique magnetic resonance tuning (MRET) as a novel sensory phenomena, based on the distance-dependent quenching displayed by the MNPs in T1 weighted MRI. As a result, the T1 MRI intensity dramatically rises as the quencher’s distances from the scanner increases. By using a target (enzyme) specific cleavable linker, this technique was applied for the sensing of biological analytes. The separation of a superparamagnetic quencher and a paramagnetic enhancer as a result of the target enzyme’s cleavage of the linker produced an increased MRI signal.
We have compiled the latest research on magnetic material-based biosensing devices in this area. The below figure presents magnetic material-based biosensing devices discussed in this chapter. Concerning the immobilized identifiable bioreceptors, they have beneficial functional qualities including their magnetic property, minimal background noise, excellent dispersions, and highly biocompatible surfaces. Modern biological devices may now be used for on-site or high-throughput applications by replacing complex monitoring biomedical equipment thanks to the ongoing interdisciplinary development of magnetic-particle-based biosensing methods. We have focused on two basic types of biosensing devices, namely optical sensing devices and electrochemical sensing devices with the integration of magnetic material for sensing biological targets, out of the many different biosensing devices that are now accessible. To provide helpful insights on point-of-care medical devices, we have focused a lot of attention on smartphones and other mobile devices that are integrated with detection in paper-based analytical devices or lateral-flow immunochromatographic assays (LFIA), as well as the interdigitated array microelectrode, screen-printed electrode, and microfluidic devices.
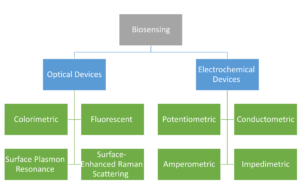
Biosensing
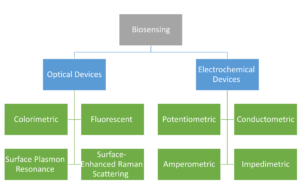
We have compiled the latest research on magnetic material-based biosensing devices in this area. The chapter’s figure presents magnetic material-based biosensing devices discussed in this chapter. Concerning the immobilized identifiable bioreceptors, they have beneficial functional qualities including their magnetic property, minimal background noise, excellent dispersions, and highly biocompatible surfaces. Modern biological devices may now be used for on-site or high-throughput applications by replacing complex monitoring biomedical equipment thanks to the ongoing interdisciplinary development of magnetic-particle-based biosensing methods. We have focused on two basic types of biosensing devices, namely optical sensing devices and electrochemical sensing devices with the integration of magnetic material for sensing biological targets, out of the many different biosensing devices that are now accessible. To provide helpful insights on point-of-care medical devices, we have focused a lot of attention on smartphones and other mobile devices that are integrated with detection in paper-based analytical devices or lateral-flow immunochromatographic assays (LFIA), as well as the interdigitated array microelectrode, screen-printed electrode, and microfluidic devices.
URL: https://link.springer.com/chapter/10.1007/978-981-99-6507-6_9
نویسنده: Mohammad-Nabil Savari
5